Which microscope has the best resolution?
When it comes to superiority in resolution, the simplest question to answer is which type of microscope achieves the highest magnification and resolution. Hands down, that is the electron microscope. In fact, the Guinness World Record for the highest resolution is held by an innovative, algorithm-driven version of the electron microscope that visualized single atoms of oxygen, scandium, and praseodymium. But does “highest” always equal “best”?
and what is “best”?
Electron microscopes shoot a concentrated beam of electrons at a target object. An image is produced as the electrons pass through the specimen and are detected. Because the electron wavelength is several thousand times shorter than that of light, the resolving power of an electron microscope is a thousand times greater than that of a light microscope.
But is it the best resolution? Highest, yes. Best? Well, that depends on what you want to do with the resolution. In biological research, time and context often matter at least as much as size, which is why superresolved light microscopy techniques like STED and MINFLUX can play to their full strength here.
The best (re)solution for your research
As you consider the advantages of resolution for your research, you should have a clear idea of what resolution means. It is distinct from magnification. In light microscopy, it is limited by an immutable property of light. And it is achieved and measured in different ways. Here are two articles to get you up to speed: “What is resolution?” and “How to measure resolution?“
Every scientific instrument comes with tradeoffs. Augmented power in one dimension, invariably curtails another. In the case of microscopes, boosting resolution complicates sample preparation and narrows the application spectrum. The best resolution is not always the highest resolution. When you’re looking for the best resolution, consider what you want to see. Most of the time, not only size but also time and context matter.
It’s no bed of roses in an electron microscope
Biological specimens require a lot of sample preparation for electron microscopy. Why? Because you’re doing the equivalent of sending them into space and then blasting them with high energy. First, the specimen must be fixed. Otherwise, the energy of the electron beam will destroy it. The specimen must also be dehydrated to survive the intense vacuum inside the microscope. Then, many biological specimens are not conductive. As a result, electrons can’t pass through the sample, and you don’t get an image. Making biological specimens conductive involves coating them with a thin layer of metal.
Clearly, the hostile environment of an electron microscope precludes working with live or unfixed samples. So, if you’re interested in the movement, changes, and context that constitute life, a slight downgrade in resolution is likely your best bet. Enter the world of super-resolution microscopy.
In life, time matters
In the realm of light microscopy, MINFLUX has repeatedly demonstrated single-digit nanometer resolution and below. The characterization of nuclear pore architecture and mitochondrial protein patterns are just two examples of the technology’s spatial resolving power. This capacity grants a completely new perspective on molecular structure in biological context, revealing the architecture of biomolecules and their interactions.
And yes, you can work with living cells.
In fact, the most distinctive feature of MINFLUX is its temporal resolution, which gives it the most advanced tracking capabilities of currently established microscopy technologies – by a long shot. That means that you can watch changes and movement happening inside living cells. The ability to differentiate events that are just a hundred microseconds apart expands the applications of MINFLUX from structural biology and slow processes like gene expression, to diffusion phenomena and even conformational changes of biomolecules. An example of this unprecedented power was the recent tracking of a kinesin-1 molecule walking along microtubules, including the corresponding configurational changes occurring at each step.The movement of kinesin-1 has never been tracked in a living cell before.
Balancing resolution, flexibility, and ease of use
Research that does not require characterizing individual molecules but rather their spatial relation to others has a broader range of microscopy technologies at its disposal. Another step down on the resolution scales makes widefield, confocal, STED, and PALM/STORM microscopy all options (see figure). As super-resolution technologies, STED and PALM/STORM outperform the spatial resolution of diffraction-limited confocal and widefield microscopy by a factor of 10. Commonly discriminating objects at 20 nm, STED is also fast, which has enabled, for example, visualizing the fission and fusion of mitochondria with exceptional clarity.
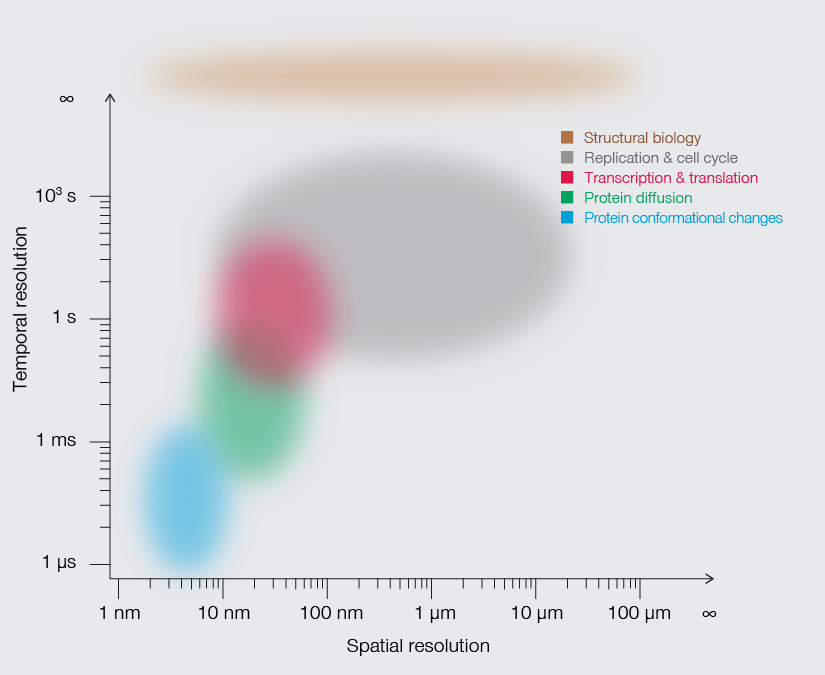
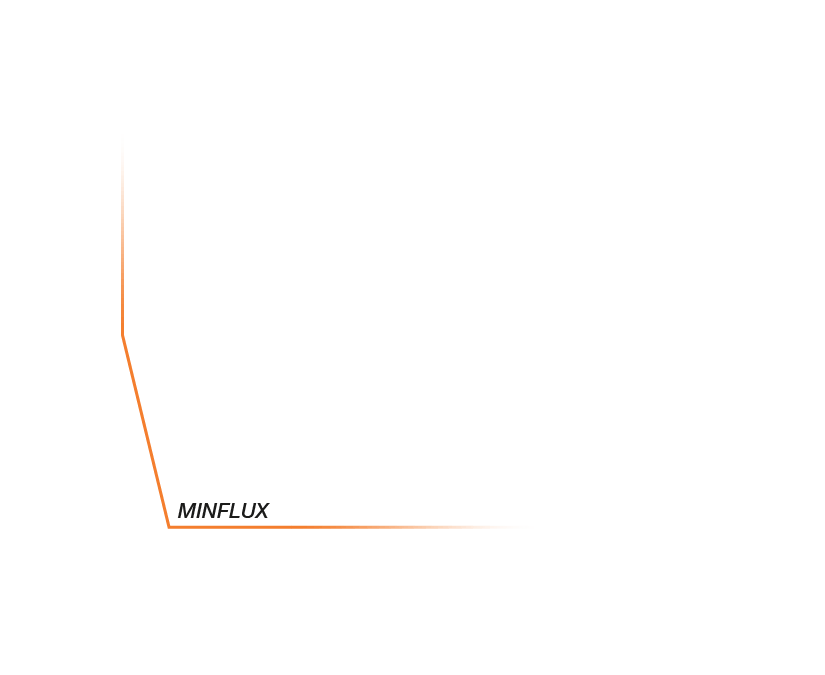
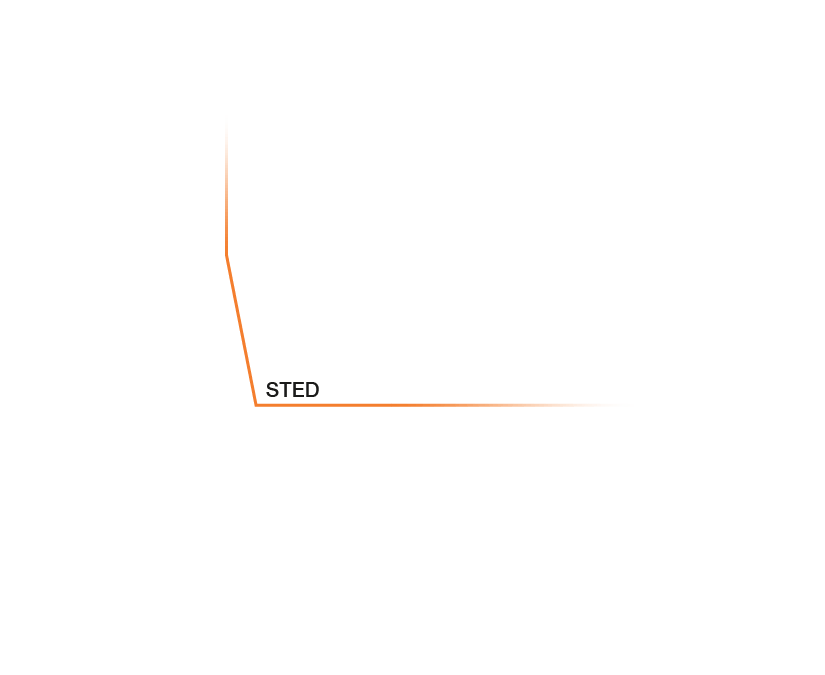
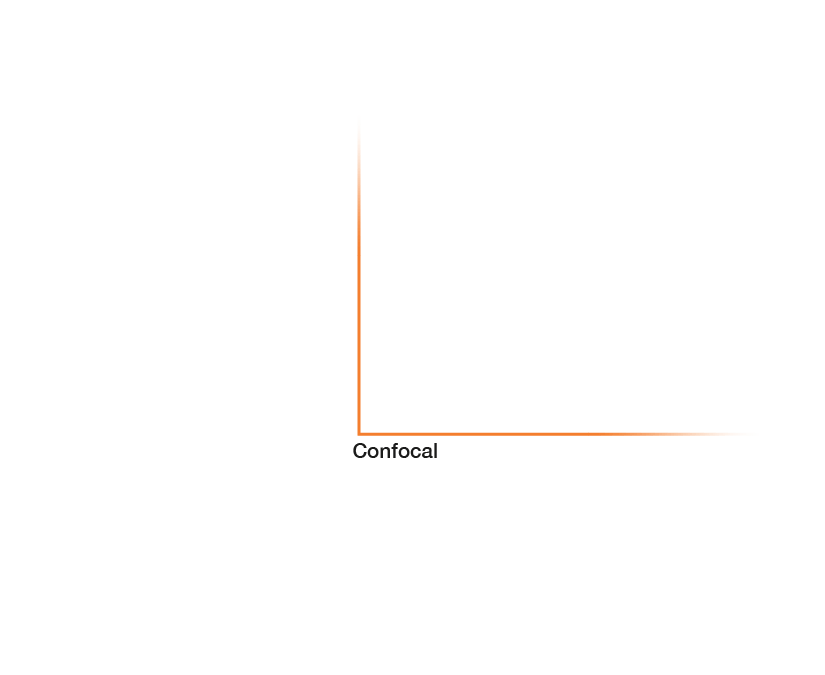
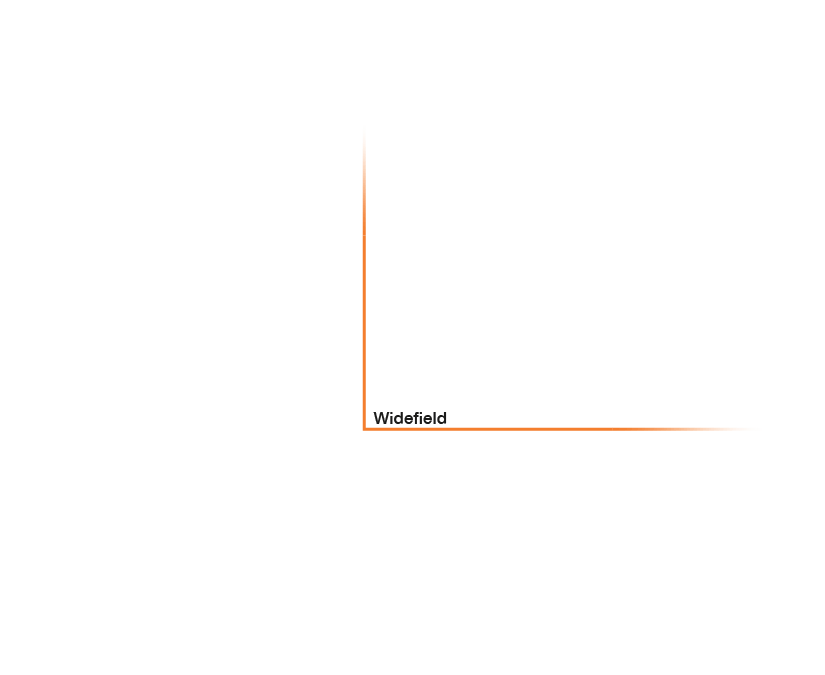
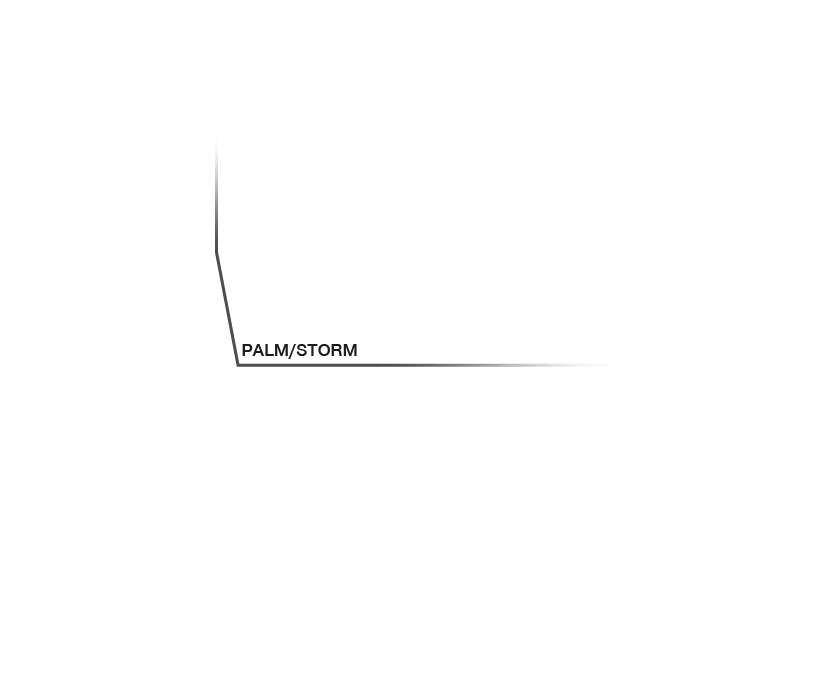
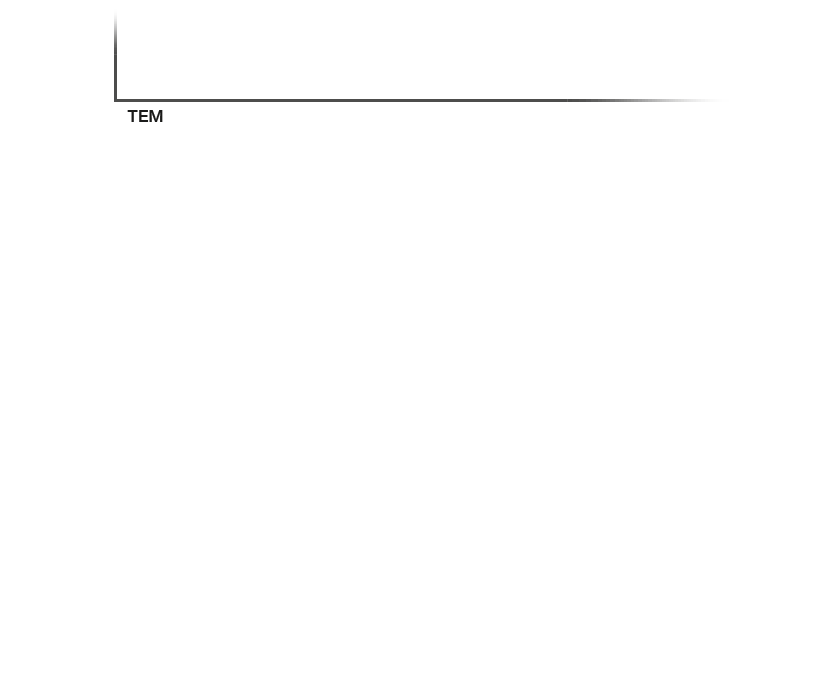
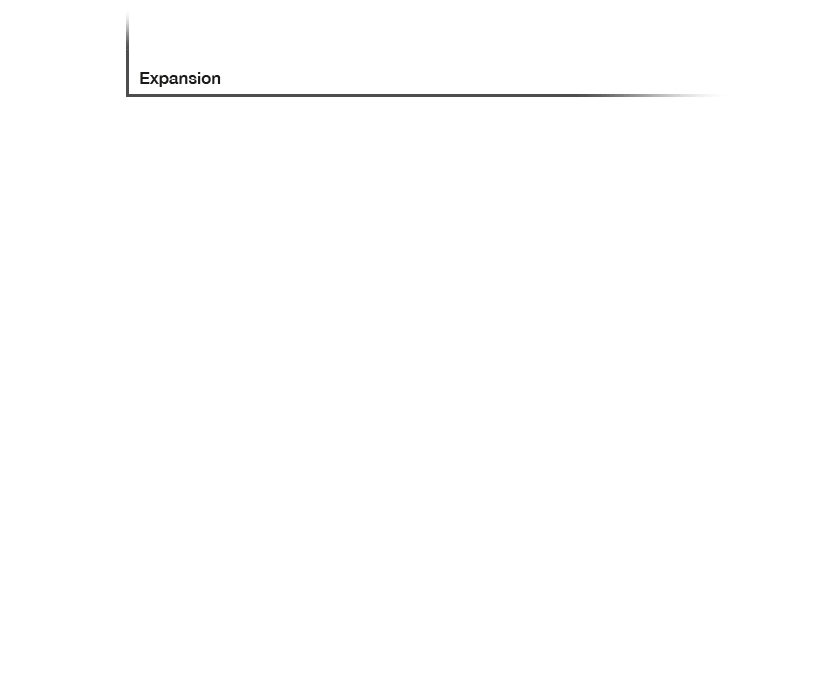
Approximate temporal and spatial resolution range of microscopy methods.
Perhaps the most important differentiators of STED are its economic photon budget and easier sample preparation and data analysis compared to PALM/STORM. In fact, as a mature technology, STED microscopes are as easy to use as standard confocal microscopes. Furthermore, STEDYCON uniquely merges all three parameters – strong resolution, intuitive usability, and broad flexibility – into one exceptional instrument: a sleek box with a favorable price tag that transforms your existing widefield microscope into a confocal and a full-fledged STED instrument.