Everything about superresolution
Have you ever asked yourself, how STED works? What’s the difference between STED, STORM, and MINFLUX? What is “targeted readout” and “stochastic readout”? How exactly does RESOLFT work? Read on to find out.
Abbe’s diffraction barrier
Challenge
If individual marker molecules (black dots below) are closer than the diffraction barrier they cannot be imaged separately – their individual blurred images overlap and all you see is an unsharp, hazy soup without any details under 200 nm.
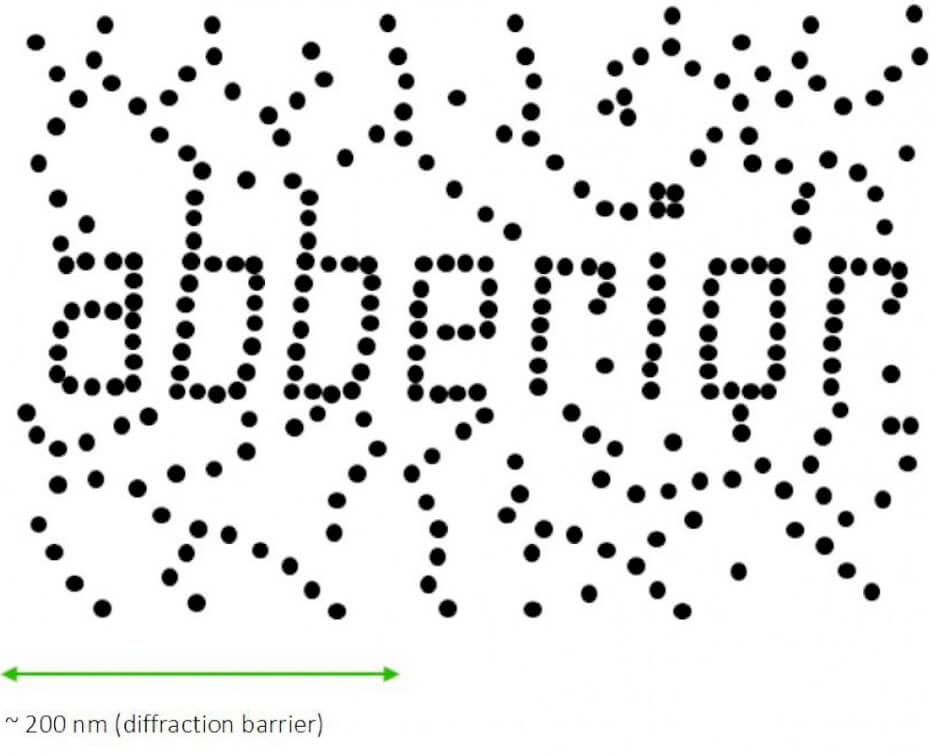
Due to diffraction, the area illuminated by a focused excitation laser (green, below) is always larger than half the wavelength of the light used. Every molecule located within this area emits light if there’s more than one there is no way they can be distinguished.
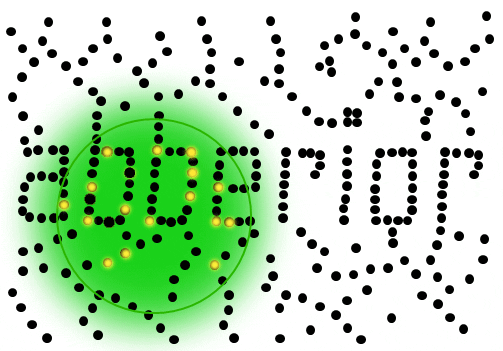
Solution
All fluorophors adjacent to a sub-diffraction-sized area are temporarily kept dark, so that they can be registered separately. This way, their blurred images do not overlap they can be recorded sequentially. The basic receipt for superresolution is indeed ingeniously simple – introduce a controllable on/off transition of the dye label.
Consequently, the dye is the key for nanoscale resolution. abberior is specialized in designing dyes with on/off transitions that can be controlled via a second light source.
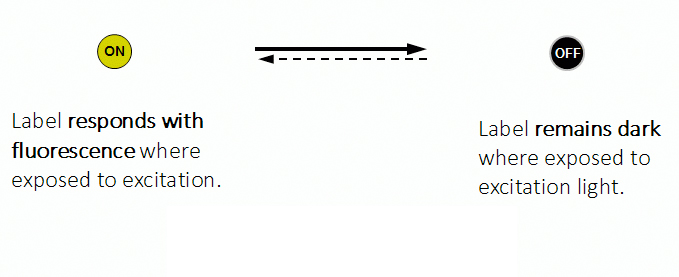
STED Stimulated Emission Depletion
Principle
STED microsocopy uses a second, red-shifted light beam. All molecules irradiated with this STED light are confined to the ground state (off-mode). The key is to modify the STED-beam in such a way that it has a tiny hole in the center where its intensity is zero, for example by giving it the well-known STED-“donut” shape. This way, all molecules except the ones in the center of the donut stay dark temporarily. Any fluorescence that is detected must necessarily come from this sub-diffraction-sized spot – their location is now known more precisely. The diffraction barrier is broken.
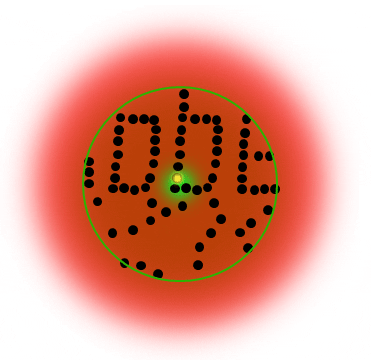
Below is a Jablonski diagram of the STED process of a fluorescent molecule. After optical excitation (1) from the ground state S0 to the first excited state S1 two ways are possible to return into the ground state: in case the molecule is in an area with STED photons, the electron gets stimulated down into the ground state and no fluorescence occurs (2). If the molecule is in the center of the donut, it returns to the ground state under emission of a fluorescence photon (3).
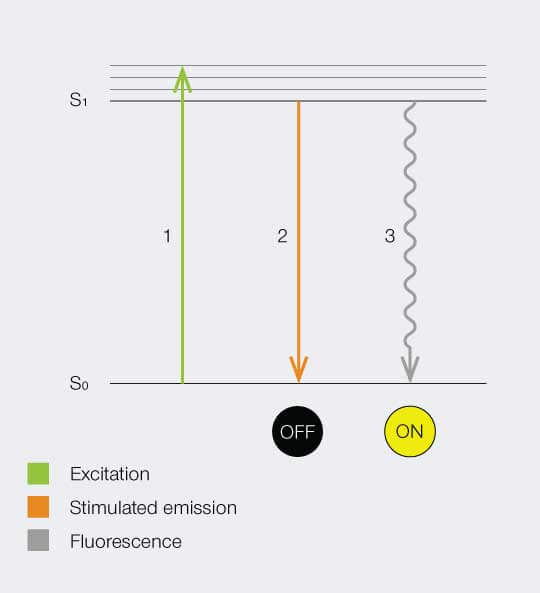
Setup
To implement the STED technique, the microscope design needs to consider a second focused light beam (STED light) besides the excitation beam. Molecules in areas subject to STED light above the saturation threshhold are forced into the off state. The phaseplate is responsible for converting the plain STED beam into a donut shape.
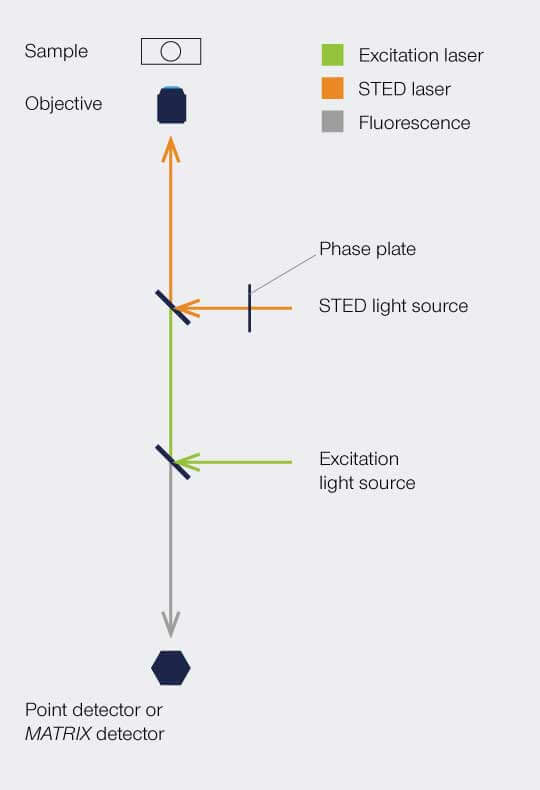
The complete image is created by scanning the overlayed focus of both beams through the sample. At each position, the signal from the very center of the focus is detected.
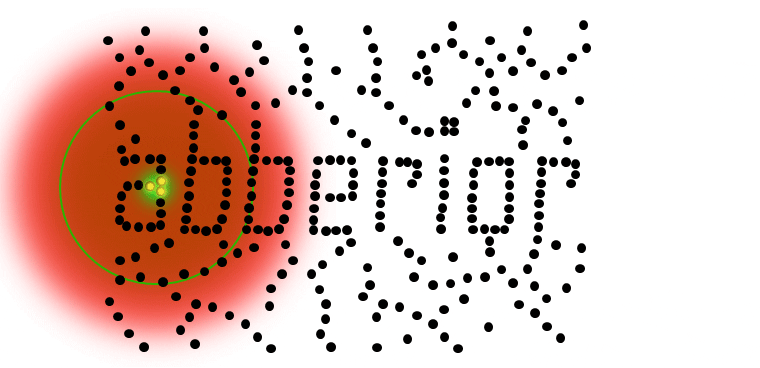
Results
STED microscopy was the first technique to abandon the diffraction barrier in optical microscopy. STED features theoretically unlimited resolution, which can be expressed by a ‘modified’ Abbe-equation:

where Δx denotes the smallest feature in space that is resolvable, λ denotes excitation wavelength and I denotes STED intensity. NA is the numerical aperture of the objective lens and I sat denotes how well the dye reacts to STED photons.
Strictly speaking, the detector only needs to see one photon to identify an individual molecule (or cluster) with nanoscale resolution. No mathematical algorithms are needed to render an image below the resolution barrier, STED is pure physics! Once molecules are separated, they can be localized even better (below).
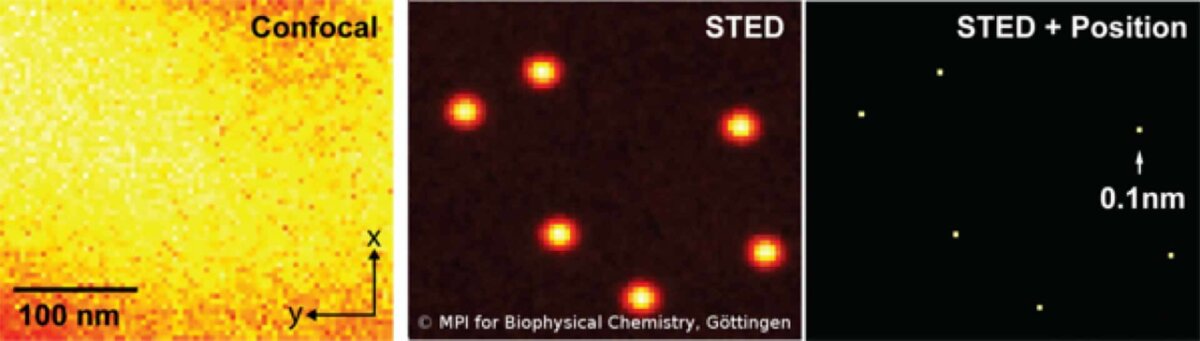
RESOLFT Reversible Saturable Optical Fluorescence Transition
Principle
RESOLFT stands for a general switching principle: the switching mechanism does no longer need to be purely electronically, but can include switching mechanisms like for example conformational changes of a molecule. Strictly speaking, the STED method is one possible implementation of the RESOLFT concept.
The approach is similar as shown for STED – a beam scanning setup with overlapping beams of the excitation and the switching beam.
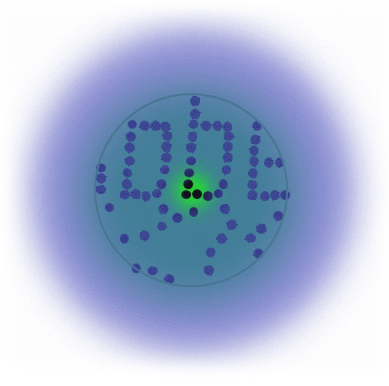
Depending on the implementation of the switch, typical saturation intensities and typical switching times are characteristic – e.g. conformational changes feature much lower switching intensities, while the switching time is much longer compared to electronical states (as for STED).
Shown below is an exemplary implementation of a RESOLFT-switch via light induced chemical reaction which generates the highly fluorescent dye from the colorless and non-fluorescent precursor.
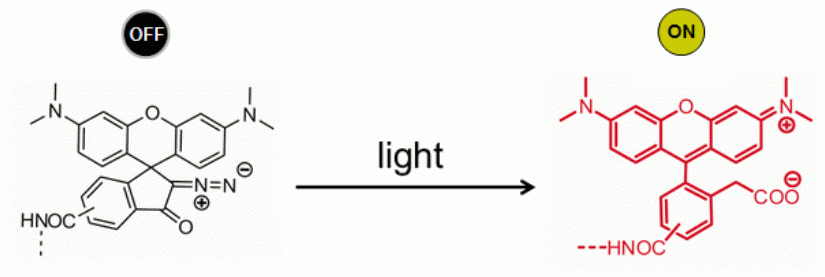
Setup
The implementation is similar as shown for STED – a beam scanning setup with overlapping beams of excitation and switching beams. In a STED setup, the switching beam would be denoted as STED beam. The phaseplate is responsible for redistributing the switching light in the focal plane, i.e. for creating the donut.
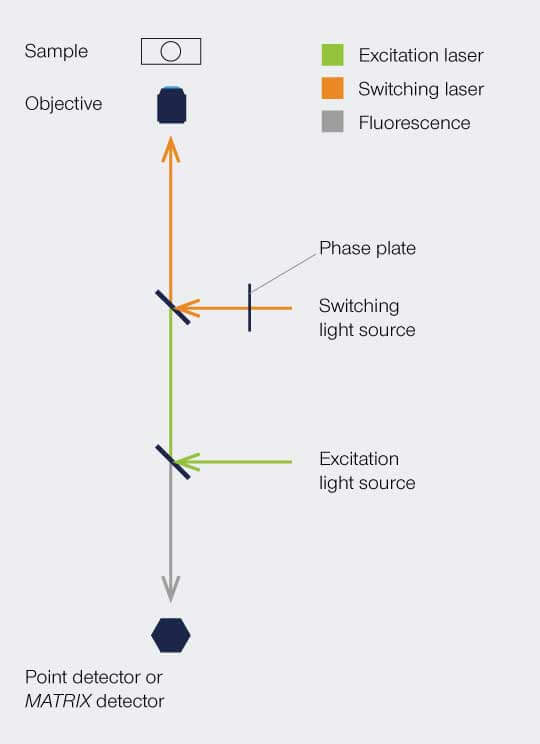
Result
RESOLFT features theoretically unlimited resolution (see equation from the STED section), since only the molecule in the center of the focus is left in the on-state.
The detector only needs to see at minimum one photon to detect individual molecule (clusters) on the nanoscale resolution.
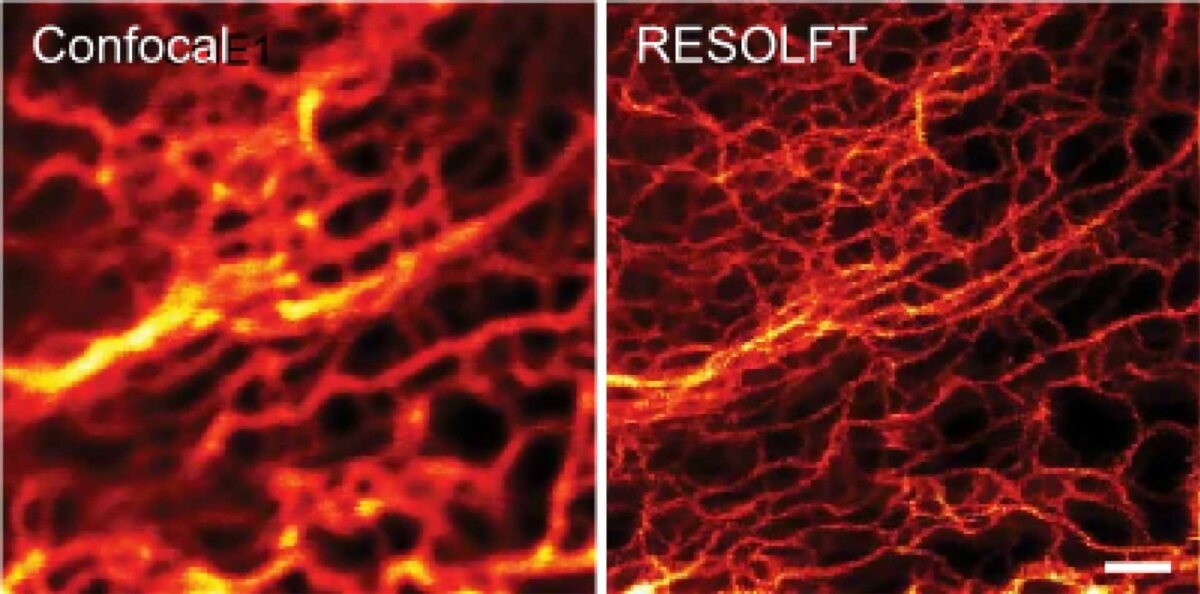
PALM Photoactivated Localization Microscopy
STORM Stochastic Optical Reconstruction Microscopy
Principle
The basic principle of PALM and STORM dictates that all molecules are prepared in the off state, then, a small subset of individual single molecules are stochastically switched into the on state. Typically, both states are realized via a photoizomerisation between molecular states (e.g. cis/trans isomerization)
After precise readout of the location of each individual molecule, they are switched back to the dark state or bleached with readout light. Sequential readout of stochastically switched molecule subsets results in a high resolution image of the label distribution.
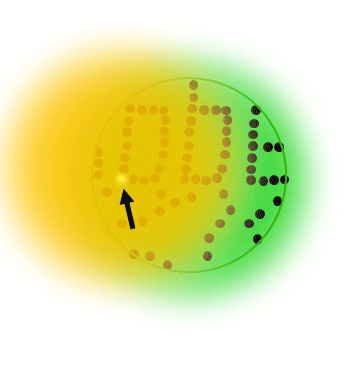
Setup
PALM and STORM implement a stochastic readout of the molecule positions. As such, the image is taken iteratively by overlapping all identified positions.
On the implementation side, a PALM or STORM microscope is quite simple: a widefield-setup with a single-photon sensitive area detector.
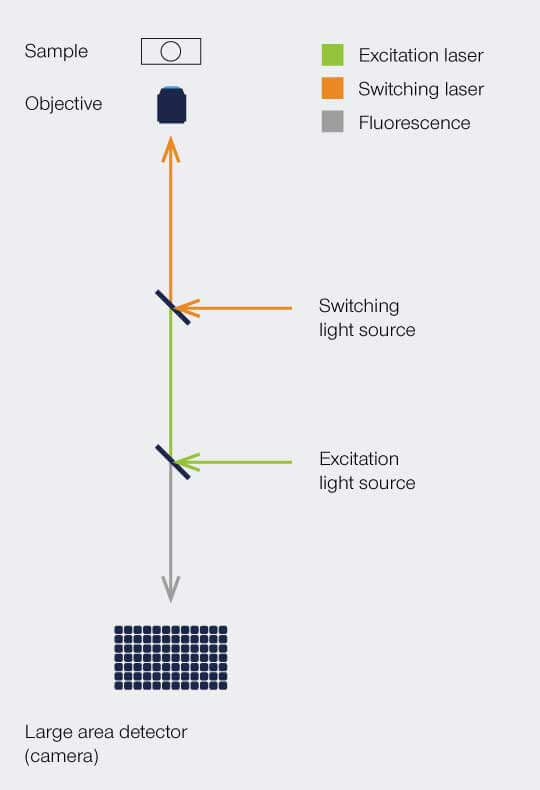
Results
The PALM and STORM methods provide theoretically unlimited localization accuracy of individual dye (clusters). The localization accuracy depends on the number of photons (N) being emitted by one dye (cluster):

where Δx denotes the localization accuracy of each individual molecule, λ denotes the excitation wavelength, and NA is the numerical aperture of the objective lens.
To deliver a high-resolution image the PALM and STORM concept must leverage numerical methods to calculate and overlay the identified positions of individual molecules.