STED and confocal microscopy
Since the 1990s, confocal microscopes have been a staple in labs visualizing biological or material specimens. However, around 2010, the scientific literature showed a distinctive rise in the use of superresolution microscopy, with STED leading the way. That development naturally prompted the question: how does the established confocal microscope compare to the (now not so) “new kid on the block”? Hint: you should (and can) use both.
the fraternal twins
In fact, there is not the one reason as to why a confocal microscope beats out classical wide-field; it’s a little bit of everything. The resolution of a confocal microscope is certainly higher, but most importantly, optical sectioning is significantly improved. Optical sectioning is the ability to generate a clear image of the focal plane – i.e. the plane in the sample the microscope’s objective lens is focused on – by suppressing signal that comes from out-of-focus areas.
The best way to think of optical sectioning is to imagine a simple sample structure, such as two cell membranes lying on top of each other. If one membrane lies exactly in the focal plane of the microscope, we should in principle be able to observe it brightly and sharply. But what about the second one, which is out of focus? Without optical sectioning, the blurred light coming from this defocused membrane would add to the focused light of the first membrane. We would see both at the same time, unable to distinguish them. With perfect optical sectioning, in contrast, the out-of-focus membrane would be completely dark, greatly reducing the background in our image.
A comparison of confocal and STED microscopy
A side-by-side comparison, as summarized in the table , highlights differences between the two methods that can impact their appropriateness for different applications. At abberior, however, we find this comparison to be moot. For us, both technologies have their rightful place in scientific inquiry. In fact, the two are inextricably connected. What makes out a STED system is the use of a donut-shaped light beam – the STED beam – in addition to the excitation beam. Take the STED beam away, and you’re left with a standard confocal microscope.
With focused illumination, high-intensity fluorescent signal, and pinhole filtering, a confocal microscope produces exceptional optical sectioning and 3D reconstructions of suitable samples (see Optical sectioning, or: tackling the background problem).
STED microscopy does the same, but with up to 10x higher resolution.
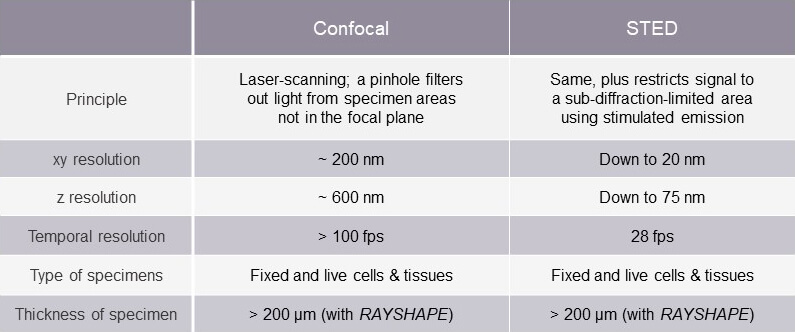
The superresolution revolution
The commercialization of STED microscopy and other superresolution methods marks the advent of the era of nanoscopy, a time when not only the structure but also the dynamics of life can be examined in unprecedented detail by light microscopy. STED has revealed a sub-diffractive world of biological structures that confocal microscopes could never discriminate, and it can be applied to living systems. Researchers now visualize the striped pattern of ßIV-spectrin in axons, the interaction of SNAP25 with other proteins of the synaptic vesicle fusion machinery, the intricate cristae of mitochondria as they split and fuse, and the ultrastructure of the cytoskeleton, gut microvilli, and inner ear hair cells.
How does STED do this?
As mentioned above, a STED system is a standard confocal microscope using two superimposed light beams. The first excites fluorescent probes to emit photons, and like in a confocal microscope, its light diffracts over an area of about 200 nm, making structures smaller than this indistinguishable. In comes the donut-shaped second beam. Wherever the intensity of that beam is non-zero, it forces excited molecules back to their ground state, essentially ‘silencing’ them. Molecules in the central “donut hole”, where there is no STED light, are unaffected and emit detectable photons (read here how STED works in more detail). Thus, STED uses the donut-shaped beam to restrict emitting molecules to an area significantly smaller than 200 nm and you get sub-diffractive resolution.Tune thepower of the STED beam to zero, and you get a normal confocal microscope with diffraction-limited resolution.
The amount of STED action mediates a continuum between the two methods: confocal microscopy is diffraction-limited, STED microscopy is not. Everything in between is possible: from no STED at all, over using just a little bit of gentle low-power STED to gain a, say, 3-fold resolution increase with hardly any impact on the sample, and up to super-high superresolution (albeit at somewhat lower speeds and signal).
Sparking shared innovation
More compelling than pitting STED against confocal microscopy is the realm of opportunities that have emerged from the continued evolution of STED. While the gain in resolution by STED is an undeniable boon, the development and improvement of components and analytics for abberior STED systems has been a hotbed of innovations that excite not only our STED evangelists but also our confocal die-hards. Enhancements and breakthroughs to improve STED microscopy also solve problems shared with confocal microscopes. Here are some examples.
RAYSHAPE: where aberrations meet correction
Biological specimens are often heterogeneous. We then pack that heterogeneity in an embedding medium, blast it with light through an immersion medium, and are disappointed when our confocal or STED microscope can’t produce the quality image we envisioned. The mismatched refractive indices of various media and the heterogeneity of the specimen itself cause light diversion. Our microscope’s ability to focus is thus compromised while light emitted by the fluorophores in the sample is also diverted. Together, these effects increasingly snuff out and smear signals the deeper you move into the sample. RAYSHAPE uses a deformable mirror that redirects diverted light back to where it belongs. With 140 digitally controlled actuators that precisely adjust the mirror surface within milliseconds, RAYSHAPE dynamically corrects aberrations at different depths as the focus plane moves through the specimen.
Whether confocal or STED, say bye-bye to aberrations with RAYSHAPE !
More from every photon with TIMEBOW
Fluorescence lifetime imaging uses differences in the decay rate of photon emission from fluorophores in a sample as an additional layer of information about a specimen. While lifetime imaging is an established method in standard fluorescence microscopy, its compatibility with STED microscopy is less well-known. And because we insist on going the extra mile, TIMEBOW — our module for seamless detection and analysis of lifetime data — works with our MATRIX detector (see below) to deliver accurate temporal and spatial information at once. This simultaneous recording of photon lifetime and its origin eliminates background and renders rich, high-contrast images. An upshot of that augmented information is a boost in resolution that allows you to reduce the intensity of the excitation beam of your confocal or STED microscope. So, you gain information and safeguard your specimen and probes.
Add the dimension of time to your imaging with TIMEBOW.
Detection in stereo with MATRIX
Both confocal and STED microscopes have some background when imaging thick samples. The pinhole of a confocal microscope cannot eliminate all out-of-focus signal, and STED excels at eliminating low-resolution signals in the focal plane but does little for the background. A point detector on either microscope records the in-focus signal and background together. Even sophisticated deconvolution can only partially subtract background and is prone to producing artifacts. However, an array of detectors that all “look” at the sample from slightly different angles can tease apart in-focus signal from background, just like your bifocal vision lets you distinguish objects in the foreground from those behind it. That’s MATRIX: multiple high-efficiency avalanche photodiodes (APDs) arranged in a hexagonal pattern on a single detector chip. MATRIX measures and subtracts the background of every pixel, so you get clear images with drastically reduced background levels for both confocal and STED microscopy.
See with 20 eyes instead of one with MATRIX.
Adaptive illumination with FLEXPOSURE
A fundamental tradeoff in fluorescence microscopy – especially when working with live specimens – is the damage to fluorophores and tissues from prolonged exposure to high-intensity light. However, most samples are sparse, with only limited areas relevant for visualization. So, why illuminate blank spaces? FLEXPOSURE adaptive illumination minimizes photobleaching and phototoxicity by applying light only where necessary. FLEXPOSURE encompasses different adaptive illumination methods that access structural information point-by-point in a sample using low-intensity confocal and STED probing to determine where full illumination is required. Using FLEXPOSURE reduces light exposure on a sample, facilitating live-cell experiments, high-resolution imaging, and superior signal. And FLEXPOSURE applies to both STED and confocal imaging.
Take it easy on the light dose with FLEXPOSURE.
How will you future-proof your lab?
Of course, STED microscopy is at the core of what we do. The intention of every idea we transform into enhancements, power, and elegance for our instruments is to gain clear and precise insights into the nanoscopic world. Some developments are exclusive to our superresolution systems, like the single-beam design of Easy3D STED that eliminates signal and resolution loss due to beam misalignment. Yet, the varied improvements that extend over to confocal microscopy show that the establishment of paradigm-shifting technologies like STED prompts a surge in novelty that ripples widely. In this way, our STED microscopes are not only cutting-edge superresolution systems but also first-class confocal instruments. As a user, you may freely tune the resolution to what you need: anything between 200 and 20 nm is possible.